Unlocking Longevity: The Interplay of Aging, Disease, and Potential Therapies
- Maya Gal
- Dec 16, 2023
- 14 min read
Updated: Nov 17, 2024
Written by: Maya Gal
Edited by: Laura Ann Lesley, William Jang, Neeraj Sakhrani
Illustrated by: Hutch Viscardi
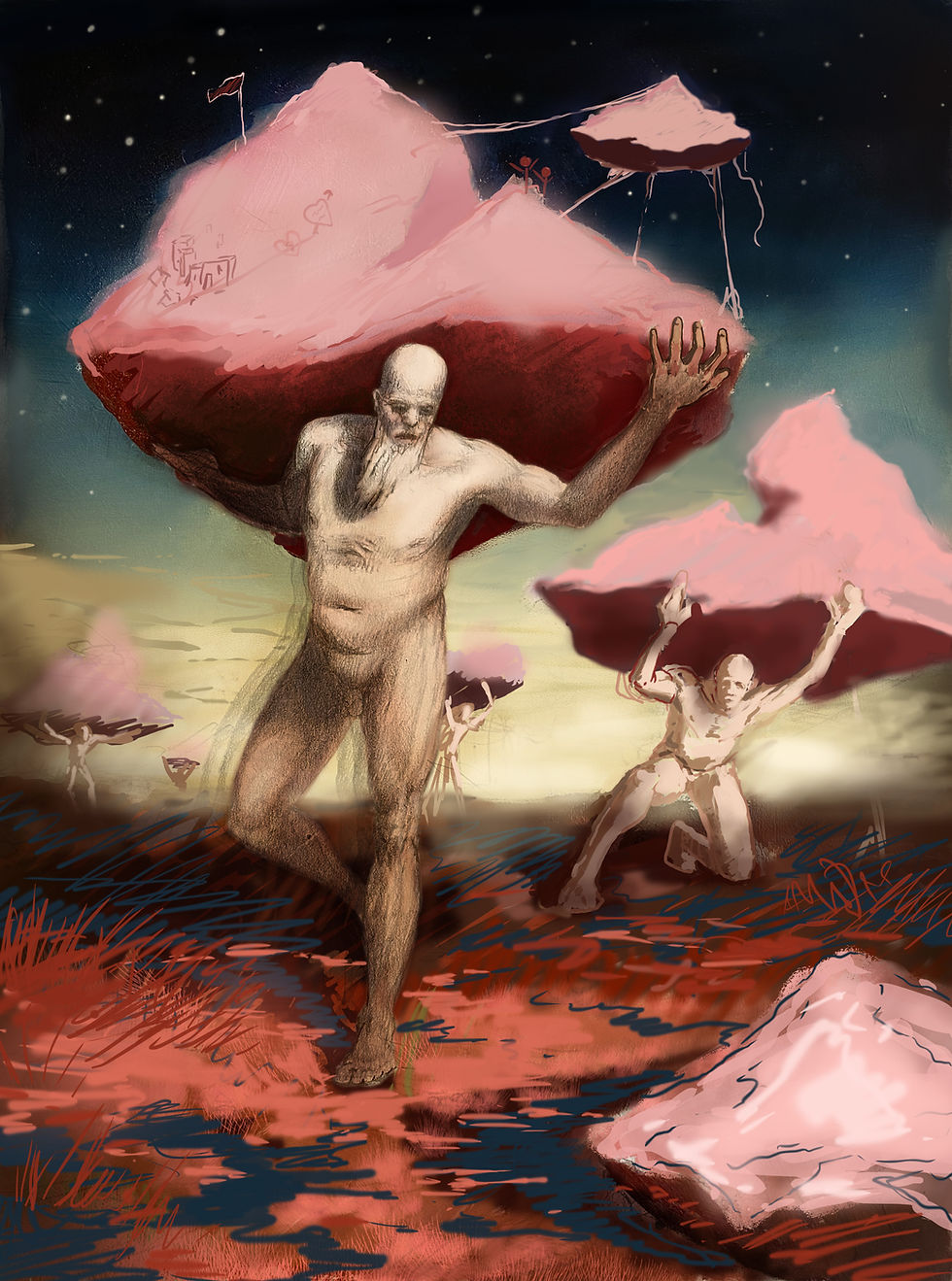
Imagine if growing older didn't mean growing sicker — that's the future scientists are working towards today. They're diving deep into the mysteries of aging, exploring why our bodies change over time and how diseases seem to follow. By unraveling the secrets of aging, we aim not for eternal life but for a life well-lived. Each discovery is a potential step toward a healthier way of growing old. With science as our ally, the golden years could become a time of health and vitality, making the journey of life even more precious!
The Intricacies of Aging: More Than Just a Number
The journey begins with understanding what aging truly entails. It's not merely the chronological passing of years but a multifaceted biological process associated with a gradual decline in bodily functions [1]. This decline dramatically heightens the risk of numerous diseases that claim millions of lives annually, such as cancer, diabetes, heart disease, and neurodegenerative disorders [1]. The societal and financial burdens imposed by these conditions are immense, underscoring the urgency of unraveling the complexities of aging.
One groundbreaking perspective is the theory of aging as a progressive loss of information [2]. This concept, deeply rooted in information theory, postulates that aging results from the gradual loss of genetic and epigenetic–modifications to our genetic code that influence gene expression– information within our cells. Over time, this information erosion disrupts cellular function and integrity, leading to phenotypic changes characteristic of aging [2]. Expanding on the concept that aging contributes to functional decline, several hallmarks have been identified, such as genomic instability, which involves DNA damage or breakage; telomere attrition, the deterioration of the protective ends of DNA during replication; and altered intercellular communication, each playing a role in the aging process [3]. These changes aren't just about wrinkles or gray hair; they're about how well our organs work, how we heal after injuries, and even how we fight off diseases.
Biological vs. Chronological Age: Predicting Mortality
Chronological age, the simple tally of years lived, doesn't fully capture the biological complexities of aging. Instead, researchers have turned their focus to "biological age," a measure that reflects an individual's physiological state more accurately [4, 5]. Biological age is assessed using various biomarkers and has been found to be a more reliable predictor of mortality and age-related disease onset than chronological age [5]. These biomarkers include modifications to DNA structure and stability and cellular efficiency [4]. Several different biological clocks have been developed with one or several of these biomarkers, and many have been shown to be reliable predictors of mortality or disease [5].
The impact of lifestyle and environmental factors on biological age is profound. Kang et al. (2018) and Wu et al. (2021) have demonstrated that certain lifestyle choices, such as regular physical activity and a balanced diet, can positively influence biological age, thereby extending the health span — the portion of one's life spent in good health [6, 7]. These findings highlight the potential for individuals to influence their aging processes through informed lifestyle choices.
Geroscience: Pioneering the Path to Enhanced Longevity
The field of geroscience, which explores the relationship between aging and chronic diseases, has seen remarkable advancements [8]. Researchers are actively developing anti-aging interventions, including drugs, supplements, and therapies, aimed at slowing the aging process and mitigating associated diseases.
Pharmacological strategies have shown considerable promise. For instance, Soo et al. (2020) discuss the potential of the medication rapamycin in extending lifespan and counteracting age-related physiological changes [9]. This compound has demonstrated lifespan-extending properties in various animal models, but its effectiveness in humans is still under investigation [9]. Similarly, Moskalev et al. (2022) highlight the anti-aging potential of another medication, metformin [10]. Like rapamycin, metformin has shown promise in animal models for extending lifespan and needs further exploration for its applicability in humans [10]. Liu (2022) also discusses the potential of both rapamycin and metformin in combating age-related changes [11].
Beyond pharmaceuticals, natural compounds and dietary supplements are also being investigated for their anti-aging potential. Various natural compounds with anti-aging properties have been identified, highlighting the need for further research to substantiate these claims and determine appropriate dosages and administration protocols. One example of a natural compound that has been studied for its anti-aging properties is astaxanthin [12]. Astaxanthin is a reddish-orange pigment, naturally synthesized by numerous microalgae, yeasts, and bacteria [11]. It’s known for its strong antioxidant properties (neutralizing unstable molecules that damage cells), and has been reported to be a more significant antioxidant agent than other antioxidants, such as vitamin C, vitamin E, and β-carotene [11].
Therapeutic approaches to anti-aging extend beyond pills. They encompass broader strategies like stem cell therapy, hormone replacement therapy, and even sophisticated genetic and cellular interventions [13-16]. These therapies aim to repair or replace damaged cells, tissues, or organs, addressing aging at the molecular or systemic level.
The Lifestyle Factor: Diet, Exercise, and Mental Well-Being
The evidence is clear: altering our lifestyle, particularly through diet and physical activity, is not just beneficial but essential for a healthier and longer life. Take intermittent fasting—it does more than just suggest health improvements; it actively bolsters cardiovascular function, sharpens the mind, and is a key to longevity, as consistently demonstrated in animal studies [17]. Similarly, the ketogenic diet, consisting of low carbs and high fat intake, is not merely a fad; it has been shown to enhance memory and increase lifespan in mice [18].
When it comes to physical activity, the case is just as compelling. Resistance exercise isn't just helpful; it's critical for maintaining muscle mass and function, which are indispensable for healthy aging [19]. Studies prove that regular exercise is fundamentally linked to retaining physical and cognitive functions in older adults, showcasing a systemic anti-aging effect [19, 20].
As for mental well-being and cognitive stimulation, their importance cannot be overstated. Engaging in mentally stimulating activities and nurturing strong social connections don't just correlate with a sharper mind—they actively foster better cognitive function as we age [21]. Research doesn't just associate these factors with cognitive health; it reveals that they are a powerful defense against the decline, significantly reducing the risk of mild cognitive impairment [21, 22]. In essence, the path to a vibrant old age is not a passive one; it's carved actively by the choices we make today.
Aging and Disease: Unraveling the Complexities and Potential Therapeutic Strategies
The intricate dance between aging and disease is one of the most profound and complex areas of current biomedical research. As we age, the risk of developing various diseases increases significantly [1]. The following section delves deeper into the underlying molecular mechanisms of aging and how they contribute to the onset and progression of several major diseases, as well as potential therapeutic strategies that target these aging processes. Understanding aging as a dynamic process that contributes significantly to disease development enables us to create treatments that focus on slowing down or even stopping the aging process itself, thereby potentially delaying or preventing the advancement of disease.
Cancer: The Interplay of Aging and Oncogenesis
Cancer, a disease characterized by uncontrolled cell growth and proliferation, becomes increasingly prevalent with age [23]. One primary reason for this is the accumulation of genetic mutations over time, which can disrupt cellular processes that normally prevent tumor development. For instance, telomeres, the protective caps on the ends of our chromosomes, naturally shorten as we age. This shortening can lead to genomic instability, a condition characterized by a high frequency of mutations within the genome of a cellular lineage. These mutations can induce genetic instability which increases cancer risk [23].
Moreover, the aged microenvironment, characterized by increased inflammatory signals, can promote the progression of cancerous cells [24]. The process of senescence, a state in which cells cease to divide but do not die, contributes to this inflammatory environment and is associated with both aging and cancer progression [24]. Therapeutically, understanding the role of these aging mechanisms in cancer has led to the exploration of novel treatments. For example, senolytics are drugs that selectively induce death in senescent cells [25]. By targeting these cells, senolytics could potentially reduce the pre-tumorigenic environment, which refers to conditions that exist prior to the initial formation of a tumor [25].
Neurodegenerative Diseases: Aging and Cognitive Decline
Neurodegenerative diseases, including Alzheimer's and Parkinson's diseases, are characterized by the progressive loss of structure and function of neurons [26]. Age is the greatest risk factor for these conditions, with several aging-related mechanisms contributing to their onset and progression. Protein aggregation, a hallmark of many neurodegenerative diseases, involves the accumulation of misfolded proteins that disrupt neuronal function. This issue is compounded by the aging body's decreased ability to clear these protein aggregates through processes like autophagy, the cellular "waste disposal" system. This decrease in autophagy can lead to an accumulation of these harmful proteins, which can disrupt cellular function and contribute to neurodegenerative diseases [26].
Mitochondrial dysfunction, another consequence of aging, also plays a critical role in neurodegeneration [27]. As the primary energy producers in the cell, mitochondria are crucial organelles for neuronal function. With age, these organelles become less efficient, contributing to the energy deficit observed in many neurodegenerative diseases [27].
While these revelations paint a concerning picture, they also lay the groundwork for targeted therapeutic strategies. Enhancing autophagy and mitochondrial function isn't just a theoretical approach; it's becoming a tangible and promising avenue to slow or prevent neurodegenerative diseases [27]. In tandem, lifestyle interventions like cognitive and physical exercises have moved beyond mere recommendations to recognized strategies that actively fortify the brain against age-related decline, underscoring the potential of these practices in fostering neural plasticity and resilience [28, 29].
Diabetes: Insulin Resistance and Aging
Type 2 diabetes is a condition where the body either doesn't produce enough insulin or can't effectively use the insulin it produces, known as insulin resistance [30]. Aging is associated with an increased risk of type 2 diabetes, largely due to increasing insulin resistance and decreased pancreatic beta-cell function, the insulin factory cells [30].
Calorie restriction and exercise have been shown to improve insulin sensitivity and are effective strategies for diabetes prevention and management [31]. Interestingly, drugs like metformin, commonly used to treat type 2 diabetes, are also being studied for their potential anti-aging effects, given their ability to mimic some of the cellular effects of calorie restriction [32].
Cardiovascular Diseases: The Heart of Aging
Cardiovascular diseases, such as heart disease and stroke, are the leading causes of death globally, with their prevalence closely linked to the aging process [33, 34]. Aging brings about several physiological alterations that play a significant role in the development of key risk factors for these diseases: high blood pressure, high cholesterol, and atherosclerosis [34].
As we age, our blood vessels naturally become stiffer and less pliable, which leads to an increase in blood pressure and a gateway to various cardiovascular issues [35]. Additionally, the aging process is accompanied by a rise in oxidative stress (overproduction of unstable molecules causing cellular damage) and inflammation, conditions that not only worsen but are also fundamental in the formation of atherosclerosis [35]. Atherosclerosis is characterized by the buildup of fatty deposits on arterial walls, which can lead to vascular blockages [34]. The relationship between these age-related changes and cardiovascular health is a critical area of study, as it holds the key to potentially delaying or preventing the onset of heart disease by targeting the aging process itself [35].
Lifestyle interventions, including a healthy diet and regular physical activity, are effective strategies for preventing and managing cardiovascular diseases [36]. Additionally, several pharmacological interventions, such as statins, a drug that is useful for cholesterol management, and antihypertensive drugs for blood pressure control, are fundamental [37, 38]. Emerging research is also exploring the potential of anti-aging therapies to target age-related mechanisms contributing to cardiovascular diseases. For instance, compounds that induce the Reperfusion Injury Salvage Kinases (RISK) and the Survivor Activating Enhancement Factor (SAFE) pathways are being investigated [39]. When these biochemical pathways are activated, they can reduce the damage caused by ischemia/reperfusion (I/R) injury, which are major contributors to heart attacks [39].
Understanding the complex relationship between aging and these diseases not only sheds light on why these conditions become more prevalent as we grow older but also opens avenues for innovative therapies that target the aging processes themselves. By focusing on the biology of aging, we may be able to develop interventions that delay, prevent, or even reverse age-related diseases, significantly improving healthspan and quality of life.
Ethical Considerations and the Horizon of Anti-Aging Research
While the scientific community strides toward understanding and potentially manipulating the aging process, significant ethical considerations arise. Partridge et al. (2009) and Small (2002) discuss the social, personal, and ethical implications of extended human lifespan, including concerns about resource allocation, healthcare costs, and the very definition of a "natural" human lifespan [40, 41].
The equity in access to anti-aging interventions also poses a profound ethical dilemma. Should these potentially life-extending treatments be reserved for the privileged, further intensifying the discrepancies that exist within healthcare, or should they be universally accessible [42]? This question becomes increasingly pertinent as we advance in scientific capabilities. Moreover, the potential impact on global population dynamics cannot be overlooked. A substantial increase in human lifespan could exacerbate existing challenges related to overpopulation, including heightened demand for resources like food, water, and energy, and intensified environmental stress [40, 41].
Despite these challenges, the future of anti-aging research holds immense promise. The development of innovative therapies that could not only extend lifespan but also mitigate morbidity — reducing the time spent in disease-afflicted states — is on the horizon. As Hodgson et al. (2020) and Yabluchanskiy et al. (2018) suggest, these advancements in geroscience could revolutionize our approach to chronic diseases, shifting the focus from treatment to prevention and healthy aging [43, 8].
The potential to significantly delay, or even reverse, aging-related decline through molecular and cellular interventions is tantalizing. However, as Ungvari and Adany (2021) cautioned, the translation of these scientific discoveries into public health practice requires careful consideration [44]. The integration of anti-aging strategies into healthcare must be guided not only by scientific evidence but also by ethical, social, and policy considerations.
In conclusion, the interplay between aging and disease is intricate and multifaceted. The promise of geroscience lies in its potential to transform our understanding of this relationship, paving the way for interventions that could enhance both the length and quality of human life. However, the path forward is rife with scientific, ethical, and practical challenges that must be navigated with care, foresight, and an inclusive perspective that considers the broader implications for society at large. The quest to unlock the secrets of aging is not just a scientific endeavor but a deeply human one, holding the promise of extended health and vitality for all.
References
[1] Niccoli, T., & Partridge, L. (2012). Ageing as a risk factor for disease. Current Biology, 22(17), R741–R752. https://doi.org/10.1016/j.cub.2012.07.024
[2] Lu, Y.R., Tian, X. & Sinclair, D.A.(2023) The Information Theory of Aging. Nature Aging 3, 1486–1499 . https://doi.org/10.1038/s43587-023-00527-6
[3] López-Otín, C., Blasco, M. A., Partridge, L., Serrano, M., & Kroemer, G. (2023). Hallmarks of aging: An expanding universe. Cell, 186(2), 243–278. https://doi.org/10.1016/j.cell.2022.11.001
[4] Ferrucci, L., Gonzalez-Freire, M., Fabbri, E., Simonsick, E., Tanaka, T., Moore, Z., Salimi, S., Sierra, F., & de Cabo, R. (2020). Measuring biological aging in humans: A quest. Aging cell, 19(2), e13080. https://doi.org/10.1111/acel.13080
5] Fransquet, P. D., Wrigglesworth, J., Woods, R. L., Ernst, M. E., & Ryan, J. (2019). The epigenetic clock as a predictor of disease and mortality risk: a systematic review and meta-analysis. Clinical epigenetics, 11(1), 62. https://doi.org/10.1186/s13148-019-0656-7
[6] Kang, Y. G., Suh, E., Lee, J. W., Kim, D. W., Cho, K. H., & Bae, C. Y. (2018). Biological age as a health index for mortality and major age-related disease incidence in Koreans: National Health Insurance Service - Health screening 11-year follow-up study. Clinical interventions in aging, 13, 429–436. https://doi.org/10.2147/CIA.S157014
[7] Wu, J. W., Yaqub, A., Ma, Y., Koudstaal, W., Hofman, A., Ikram, M. A., Ghanbari, M., & Goudsmit, J. (2021). Biological age in healthy elderly predicts aging-related diseases including dementia. Scientific reports, 11(1), 15929. https://doi.org/10.1038/s41598-021-95425-5
[8] Yabluchanskiy, A., Ungvari, Z., Csiszar, A., & Tarantini, S. (2018). Advances and challenges in geroscience research: An update. Physiology international, 105(4), 298–308. https://doi.org/10.1556/2060.105.2018.4.32
[9] Soo, S. K., Rudich, P. D., Traa, A., Harris-Gauthier, N., Shields, H. J., & Van Raamsdonk, J. M. (2020). Compounds that extend longevity are protective in neurodegenerative diseases and provide a novel treatment strategy for these devastating disorders. Mechanisms of ageing and development, 190, 111297. https://doi.org/10.1016/j.mad.2020.111297
[10] Moskalev, A., Guvatova, Z., Lopes, I. A., Beckett, C. W., Kennedy, B. K., De Magalhaes, J. P., & Makarov, A. A. (2022). Targeting aging mechanisms: pharmacological perspectives. Trends in endocrinology and metabolism: TEM, 33(4), 266–280. https://doi.org/10.1016/j.tem.2022.01.007
[11] Liu J. K. (2022). Antiaging agents: safe interventions to slow aging and healthy life span extension. Natural products and bioprospecting, 12(1), 18. https://doi.org/10.1007/s13659-022-00339-y
[12] Bjørklund, G., Shanaida, M., Lysiuk, R., Butnariu, M., Peana, M., Sarac, I., Strus, O., Smetanina, K., & Chirumbolo, S. (2022). Natural Compounds and Products from an Anti-Aging Perspective. Molecules (Basel, Switzerland), 27(20), 7084. https://doi.org/10.3390/molecules27207084
[13] Garay R. P. (2023). Recent clinical trials with stem cells to slow or reverse normal aging processes. Frontiers in aging, 4, 1148926. https://doi.org/10.3389/fragi.2023.1148926
[14] Li, Z., Zhang, Z., Ren, Y., Wang, Y., Fang, J., Yue, H., Ma, S., & Guan, F. (2021). Aging and age-related diseases: from mechanisms to therapeutic strategies. Biogerontology, 22(2), 165–187. https://doi.org/10.1007/s10522-021-09910-5
[15] Prašnikar, E., Borišek, J., & Perdih, A. (2021). Senescent cells as promising targets to tackle age-related diseases. Ageing research reviews, 66, 101251. https://doi.org/10.1016/j.arr.2020.101251
[16] Szabó, R., Hoffmann, A., Börzsei, D., Kupai, K., Veszelka, M., Berkó, A. M., Pávó, I., Gesztelyi, R., Juhász, B., Turcsán, Z., Pósa, A., & Varga, C. (2021). Hormone Replacement Therapy and Aging: A Potential Therapeutic Approach for Age-Related Oxidative Stress and Cardiac Remodeling. Oxidative medicine and cellular longevity, 2021, 8364297. https://doi.org/10.1155/2021/8364297
[17] de Cabo, R., & Mattson, M. P. (2019). Effects of Intermittent Fasting on Health, Aging, and Disease. The New England journal of medicine, 381(26), 2541–2551. https://doi.org/10.1056/NEJMra1905136
[18] Newman, J. C., Covarrubias, A. J., Zhao, M., Yu, X., Gut, P., Ng, C. P., Huang, Y., Haldar, S., & Verdin, E. (2017). Ketogenic Diet Reduces Midlife Mortality and Improves Memory in Aging Mice. Cell metabolism, 26(3), 547–557.e8. https://doi.org/10.1016/j.cmet.2017.08.004
[19] Mora, J. C., & Valencia, W. M. (2018). Exercise and Older Adults. Clinics in geriatric medicine, 34(1), 145–162. https://doi.org/10.1016/j.cger.2017.08.007
[20] McKendry, J., Stokes, T., Mcleod, J. C., & Phillips, S. M. (2021). Resistance Exercise, Aging, Disuse, and Muscle Protein Metabolism. Comprehensive Physiology, 11(3), 2249–2278. https://doi.org/10.1002/cphy.c200029
[21] Lewis, N. A., Turiano, N. A., Payne, B. R., & Hill, P. L. (2017). Purpose in life and cognitive functioning in adulthood. Neuropsychology, development, and cognition. Section B, Aging, neuropsychology and cognition, 24(6), 662–671. https://doi.org/10.1080/13825585.2016.1251549
[22] Krell-Roesch, J., Vemuri, P., Pink, A., Roberts, R. O., Stokin, G. B., Mielke, M. M., Christianson, T. J., Knopman, D. S., Petersen, R. C., Kremers, W. K., & Geda, Y. E. (2017). Association Between Mentally Stimulating Activities in Late Life and the Outcome of Incident Mild Cognitive Impairment, With an Analysis of the APOE ε4 Genotype. JAMA neurology, 74(3), 332–338. https://doi.org/10.1001/jamaneurol.2016.3822
[23] Berben, L., Floris, G., Wildiers, H., & Hatse, S. (2021). Cancer and Aging: Two Tightly Interconnected Biological Processes. Cancers, 13(6), 1400. https://doi.org/10.3390/cancers13061400
[24] Morales-Valencia, J., & David, G. (2021). The Contribution of Physiological and Accelerated Aging to Cancer Progression Through Senescence-Induced Inflammation. Frontiers in oncology, 11, 747822. https://doi.org/10.3389/fonc.2021.747822
[25] Carpenter, V. J., Saleh, T., & Gewirtz, D. A. (2021). Senolytics for Cancer Therapy: Is All That Glitters Really Gold?. Cancers, 13(4), 723. https://doi.org/10.3390/cancers13040723
[26] Azam, S., Haque, M. E., Balakrishnan, R., Kim, I. S., & Choi, D. K. (2021). The Ageing Brain: Molecular and Cellular Basis of Neurodegeneration. Frontiers in cell and developmental biology, 9, 683459. https://doi.org/10.3389/fcell.2021.683459
[27] Zimmermann, A., Madreiter-Sokolowski, C., Stryeck, S., & Abdellatif, M. (2021). Targeting the Mitochondria-Proteostasis Axis to Delay Aging. Frontiers in cell and developmental biology, 9, 656201. https://doi.org/10.3389/fcell.2021.656201
[28] Chen, L., Jiao, J., & Zhang, Y. (2022). Therapeutic approaches for improving cognitive function in the aging brain. Frontiers in neuroscience, 16, 1060556. https://doi.org/10.3389/fnins.2022.1060556
[29] Mahalakshmi, B., Maurya, N., Lee, S. D., & Bharath Kumar, V. (2020). Possible Neuroprotective Mechanisms of Physical Exercise in Neurodegeneration. International journal of molecular sciences, 21(16), 5895. https://doi.org/10.3390/ijms21165895
[30] Tudurí, E., Soriano, S., Almagro, L., Montanya, E., Alonso-Magdalena, P., Nadal, Á., & Quesada, I. (2022). The pancreatic β-cell in ageing: Implications in age-related diabetes. Ageing research reviews, 80, 101674. https://doi.org/10.1016/j.arr.2022.101674
[31] Larson-Meyer, D. E., Heilbronn, L. K., Redman, L. M., Newcomer, B. R., Frisard, M. I., Anton, S., ... & Pennington CALERIE Team. (2006). Effect of calorie restriction with or without exercise on insulin sensitivity, β-cell function, fat cell size, and ectopic lipid in overweight subjects. Diabetes care, 29(6), 1337-1344. https://doi.org/10.2337/dc05-2565
[32] Kulkarni, A. S., Gubbi, S., & Barzilai, N. (2020). Benefits of Metformin in Attenuating the Hallmarks of Aging. Cell metabolism, 32(1), 15–30. https://doi.org/10.1016/j.cmet.2020.04.001
[33] Mathers, C., Stevens, G., Hogan, D., Mahanani, W. R., & Ho, J. (2017). Global and regional causes of death: patterns and trends, 2000–15. Disease Control Priorities: Improving Health and Reducing Poverty. 3rd edition. https://doi.org/10.1596/978-1-4648-0527-1_ch4
[34] Ferrucci, L., & Fabbri, E. (2018). Inflammageing: chronic inflammation in ageing, cardiovascular disease, and frailty. Nature reviews. Cardiology, 15(9), 505–522. https://doi.org/10.1038/s41569-018-0064-2
[35] Ungvari, Z., Tarantini, S., Donato, A. J., Galvan, V., & Csiszar, A. (2018). Mechanisms of Vascular Aging. Circulation research, 123(7), 849–867. https://doi.org/10.1161/CIRCRESAHA.118.311378
[36] Lanier, J. B., Bury, D. C., & Richardson, S. W. (2016). Diet and physical activity for cardiovascular disease prevention. American family physician, 93(11), 919-924.
[37] Taylor, F. C., Huffman, M., & Ebrahim, S. (2013). Statin therapy for primary prevention of cardiovascular disease. Jama, 310(22), 2451-2452. https://doi.org/0.1001/jama.2013.281348
[38] Shin, S., Song, H., Oh, S. K., Choi, K. E., Kim, H., & Jang, S. (2013). Effect of antihypertensive medication adherence on hospitalization for cardiovascular disease and mortality in hypertensive patients. Hypertension Research, 36(11), 1000-1005. https://doi.org/10.1038/hr.2013.85
[39] Díaz-Vesga, M. C., Zúñiga-Cuevas, Ú., Ramírez-Reyes, A., Herrera-Zelada, N., Palomo, I., Bravo-Sagua, R., & Riquelme, J. A. (2021). Potential Therapies to Protect the Aging Heart Against Ischemia/Reperfusion Injury. Frontiers in cardiovascular medicine, 8, 770421. https://doi.org/10.3389/fcvm.2021.770421
[40] Partridge, B., Lucke, J., Bartlett, H., & Hall, W. (2009). Ethical, social, and personal implications of extended human lifespan identified by members of the public. Rejuvenation research, 12(5), 351–357. https://doi.org/10.1089/rej.2009.0907
[41] Small R. (2002). The ethics of life expectancy. Bioethics, 16(4), 307–334. https://doi.org/10.1111/1467-8519.00291
[42] Kim, Y., Vazquez, C., & Cubbin, C. (2023). Socioeconomic disparities in health outcomes in the United States in the late 2010s: results from four national population-based studies. Archives of Public Health, 81(1), 15. https://doi.org/10.1186/s13690-023-01026-1
[43] Hodgson, R., Kennedy, B. K., Masliah, E., Scearce-Levie, K., Tate, B., Venkateswaran, A., & Braithwaite, S. P. (2020). Aging: therapeutics for a healthy future. Neuroscience and biobehavioral reviews, 108, 453–458. https://doi.org/10.1016/j.neubiorev.2019.11.021
[44] Ungvari, Z., & Adany, R. (2021). The future of healthy aging: translation of geroscience discoveries to public health practice. European journal of public health, 31(3), 455–456. https://doi.org/10.1093/eurpub/ckaa212
Comments