Turning to Salamanders: Unlocking the Answers to Brain Regeneration
- Alondra Sánchez
- Jan 21
- 9 min read
Written by: Alondra Sánchez
Edited by: Hilal Tokat, Ariel Yu, and Lilly Hal
Illustrated by: Caroline Shen
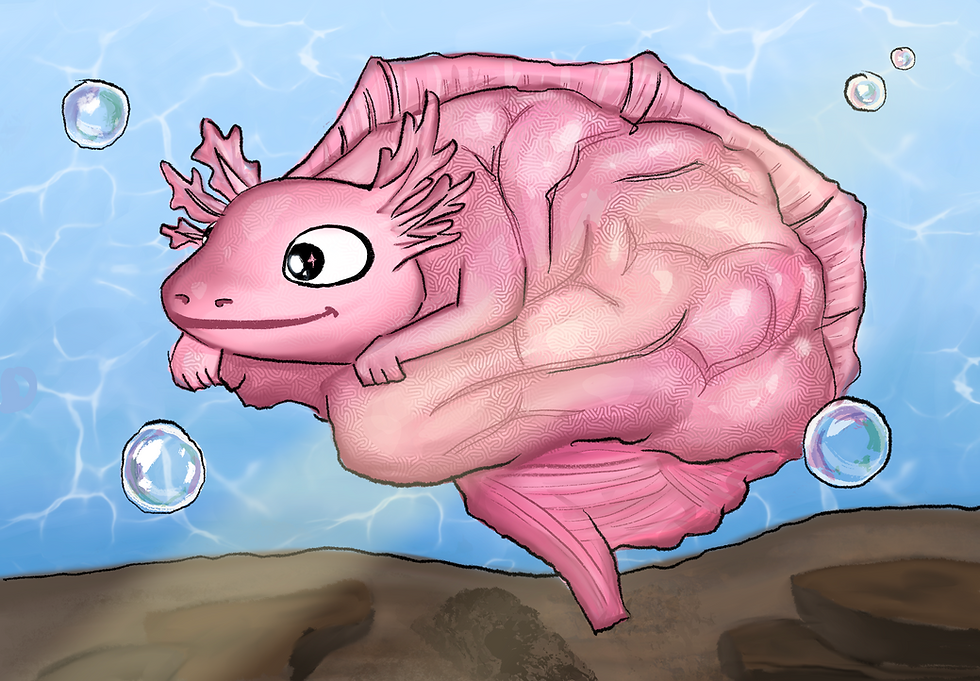
Introduction to the Axolotl
Lake Xochimilco (pronounced sow-chee-MEEL-koh), located in southern México, is home to the fascinating salamanders known as axolotls [1]. These unique creatures typically measure nine inches in length and are distinguished by their feathery external gills, nostrils, small eyelid-less eyes, and a tadpole-like fin [1]. Axolotls also have four fingers on their front limbs, five on their back limbs, and a long, slender tail, giving them their distinctive appearance [2].
What sets axolotls apart from other amphibians—and what researchers find particularly fascinating—is their remarkable ability to regrow body parts (such as their limbs, tail, jaws, heart, and even their spinal cord) after an injury regardless of their age [3]. This extraordinary trait is known as regeneration. Axolotls exhibit a remarkable ability to regenerate, but evidence suggests this efficiency may decline slightly under certain conditions, such as repeated amputations of the same limb [4]. Research shows that repeated injuries can lead to persistent misregulation of genes, including the overexpression of amphiregulin (a protein that helps cells heal and grow), which disrupts the normal regenerative process and causes delays or defects [4]. While axolotls’ regeneration remains strong overall, acknowledging these nuances provides a more accurate scientific understanding of the factors influencing regenerative efficiency. Additionally, axolotls exhibit a unique phenomenon called paedomorphosis, where they retain their juvenile characteristics throughout their entire lives. In other words, axolotls go through a process that allows them to stay “forever young.”
Brain regeneration is the process of repairing or replacing damaged brain cells, tissues, or functions, often after injury or disease [6]. Studying brain regeneration is crucial because the brain has limited natural healing abilities, making conditions like stroke, traumatic brain injury, and neurodegenerative diseases particularly devastating [7]. Understanding and enhancing this process could revolutionize treatments, improving recovery and quality of life for millions of people worldwide. Paedomorphosis may help researchers explore how damaged brain tissues in humans can return to their healthy, youthful state after traumatic brain injuries. This understanding can have the potential to advance treatments for neurological diseases and deficits. Despite existing research, the biological process behind the mechanism of paedomorphosis is only partially understood and still unclear. How it is linked to a deficiency in thyroid hormone production, which prevents full metamorphosis in axolotls by disrupting development and helps explain their retention of juvenile traits, remains an open question [8].
Another process that axolotls possess allows them to regenerate lost body parts. This process, known as epimorphic regeneration, involves specialized cells in their body reverting to a basic form, enabling them to multiply and develop into new tissues [9]. This type of regeneration, seen in axolotls, could transform medicine by teaching us how to reset human cells to a basic state, allowing them to grow into new tissues. This may pave the way for groundbreaking treatments, helping to repair injuries or regrow lost body parts, and offering hope for conditions once considered untreatable.
Regenerative Medicine
Humans don’t undergo paedomorphosis, nor do they possess the strong regenerative abilities found in axolotls. However, humans retain the ability to regenerate certain tissues, as demonstrated by wound healing and the liver's capacity to regrow [10]. This regeneration relies on the division of existing liver cells to recover the organ's function and size, rather than producing entirely new cells [11]. Unlike axolotls, humans cannot recreate identical tissue in their bodies after they have been damaged. This limitation also applies to muscle and bone repair. Muscle regeneration can restore damaged muscle fibers but cannot replace an entire muscle if it is removed. Similarly, while bones can heal fractures, they cannot fully restore themselves to their original state after injury. These limitations in exact tissue regeneration and the amount of tissue replacement pose a challenge that regenerative medicine aims to overcome [11].
So far, researchers in regenerative medicine have been able to use extracted stem cells—unspecialized cells that can develop into different types of specialized cells—as a guide to form complex structures like tissues or organs [12], but a process that they aim to achieve is to be able to have stem cells undergo epimorphic regeneration (the process of regrowing lost body parts through cellular growth and specialization). To contrast human regenerative medicine with the axolotl's epimorphic regeneration, current regenerative therapies for humans focus on using stem cells as building blocks that transform into specific tissues, rather than reworking existing tissues back into basic building blocks to create new types [13]. While human therapies use stem cells to create new tissues from undifferentiated cells, axolotls can regenerate by reverting existing cells to a basic state, allowing them to regrow complex body parts. This process in axolotls offers insight into how human cells could potentially be reset and regenerated in a similar way.
Future Outlooks
A neurological disorder termed Broca’s aphasia is a type of language disorder characterized by difficulties in speech production while comprehension remains relatively intact [14]. Broca’s aphasia, often caused by a stroke, affects about 225,000 people in the USA each year. With over 2 million people living with aphasia in the country, it is important to understand this disorder to help improve communication and support rehabilitation for those affected [15]. Since stroke is the third leading cause of death in the US, recognizing and addressing aphasia is key to enhancing the quality of life for stroke survivors [16]. This condition arises from damage primarily to Broca’s area, located in the left frontal lobe of the brain, but may also involve surrounding areas and underlying structures [17]. Damage of the Broca’s area includes lesions, scars, or abnormal tissue in the brain that are caused by traumatic brain injuries such as strokes and/or tumors [18]. Given the axolotl’s remarkable epimorphic regeneration capabilities, further exploration of this mechanism could provide regenerative medicine with innovative treatment options to repair scarred brain tissue, particularly in Broca’s area. This research holds the potential to help individuals affected by Broca’s aphasia regain their language function and improve their quality of life.
Researchers can leverage current knowledge of animal regeneration to advance studies on lesion regeneration by examining the ability of mice to regenerate parts of their feet. This can be achieved through the discovery of Bone Morphogenetic Protein (BMP) cells. BMPs are crucial proteins involved in the development of bones and cartilage, found in both humans and animals [19]. A study shows that mice can regrow their digits (toes) after amputation, with BMP cells playing a key role in the process. These cells are responsible for regenerating portions of the digits, although they do not regenerate the entire toe [20].
A promising avenue for future research involves investigating a specific type of protein (like BMPs) that is found in both animals, such as axolotls and humans, and plays a crucial role in the epimorphic regeneration process of axolotls. Understanding this protein could lead to its incorporation into clinical treatments, potentially as dosages given to aid patients struggling with Broca’s aphasia. In axolotls, BMP signaling also plays a significant role in limb regeneration, but their ability to fully regenerate complex structures like entire digits suggests that axolotls have evolved specialized mechanisms that enhance or maintain BMP signaling to support full tissue regeneration [21]. Thus, while BMP cells contribute to digit regeneration in both species, the regenerative capacity of axolotls goes far beyond that of mice, offering insights into how BMP signaling could potentially be harnessed for more extensive regeneration in humans or other animals. The connection between BMP signaling in regeneration and Broca's aphasia is more conceptual and lies in understanding the potential for tissue and neural regeneration, particularly in the context of brain injury or damage. The regenerative mechanisms observed in axolotls—such as the use of BMPs to promote tissue regeneration—can serve as a potential model for enhancing neural repair in humans, although it is important to note that while axolotls can regenerate parts of their brain, this process is not fully understood, particularly in terms of complex functional recovery. The model would likely involve manipulating BMP signaling to promote neurogenesis (growth of new neurons) and synaptogenesis (formation of new brain connections), as well as adapting cells to support tissue repair [22]. This could be achieved through gene editing, growth factor delivery, and recreating a regenerative microenvironment in the brain [22]. Ultimately, the goal would be to translate these findings into clinical treatments for conditions like aphasia by improving neural tissue regeneration and functional recovery after brain injury. In short, axolotls serve as an invaluable model organism for advancing regenerative medicine research, especially in the fields of tissue regeneration and neural repair.
While regenerative medicine holds tremendous promise for revolutionizing treatment options, particularly in areas like brain injuries and aphasia, several critical risks and obstacles must be carefully addressed. The introduction of germline editing technologies like CRISPR raises critical concerns, which include the possibility of unintended tumorigenesis due to off-target effects, which could result in harmful genetic alterations that might be passed down through generations [23]. The complexity of translating animal regenerative abilities, such as those seen in axolotls, into human clinical applications is not a straightforward process, as the mechanisms underlying their regenerative abilities are vastly different from those in humans. To ensure the safety and efficacy of these treatments, rigorous regulation, extensive preclinical and clinical testing, and a thorough evaluation of ethical concerns are essential. Only through careful oversight and a comprehensive understanding of both the biological and ethical implications can clinicians fully realize the potential of regenerative therapies to treat complex conditions while minimizing the risk of unintended consequences.
References
[1] Humphrey, R. R. (1975). The axolotl, Ambystoma mexicanum. In R. C. King (Ed.), Handbook of genetics (pp. 1-29). Springer. https://doi.org/10.1007/978-1-4613-4470-4_1
[2] Farkas, J. E., & Monaghan, J. R. (2015). Housing and maintenance of Ambystoma mexicanum, the Mexican axolotl. In A. Kumar & A. Simon (Eds.), Salamanders in regeneration research (Vol. 1290, pp. 39-46). Methods in Molecular Biology. Humana Press. https://doi.org/10.1007/978-1-4939-2495-0_3
[3] Roy, S., & Gatien, S. (2008). Regeneration in axolotls: A model to aim for! Experimental Gerontology, 43(11), 968-973. https://doi.org/10.1016/j.exger.2008.09.003
[4] Bryant, D. M., Sousounis, K., Payzin-Dogru, D., Bryant, S., Sandoval, A. G. W., Martinez Fernandez, J., Mariano, R., Oshiro, R., Wong, A. Y., Leigh, N. D., Johnson, K., & Whited, J. L. (2017). Identification of regenerative roadblocks via repeat deployment of limb regeneration in axolotls. NPJ Regenerative medicine, 2, 30. https://doi.org/10.1038/s41536-017-0034-z
[5] Bryant, D. M., Sousounis, K., Payzin-Dogru, D., et al. (2017). Identification of regenerative roadblocks via repeat deployment of limb regeneration in axolotls. npj Regenerative Medicine, 2, 30. https://doi.org/10.1038/s41536-017-0034-z
[6] Martino, G., Pluchino, S., Bonfanti, L., & Schwartz, M. (2011). Brain regeneration in physiology and pathology: the immune signature driving therapeutic plasticity of neural stem cells. Physiological reviews, 91(4), 1281–1304. https://doi.org/10.1152/physrev.00032.2010
[7] Zamproni, L. N., Mundim, M. T. V. V., & Porcionatto, M. A. (2021). Neurorepair and regeneration of the brain: A decade of bioscaffolds and engineered microtissue. Frontiers in Cell and Developmental Biology, 9, 649891. https://doi.org/10.3389/fcell.2021.649891
[8] Crowner, A., Khatri, S., Blichmann, D., & Voss, S. R. (2019). Rediscovering the Axolotl as a Model for Thyroid Hormone Dependent Development. Frontiers in endocrinology, 10, 237. https://doi.org/10.3389/fendo.2019.00237
[9] Dinsmore, C. E. (1991). A history of regeneration research: Milestones in the evolution of a science (1st ed.). Cambridge University Press.
[10] Atala, A., Irvine, D. J., Moses, M., & Shaunak, S. (2010). Wound Healing Versus Regeneration: Role of the Tissue Environment in Regenerative Medicine. MRS bulletin, 35(8), 10.1557/mrs2010.528. https://doi.org/10.1557/mrs2010.528
[11] Roy, S., & Gatien, S. (2008). Regeneration in axolotls: A model to aim for! Experimental Gerontology, 43(11), 968-973. https://doi.org/10.1016/j.exger.2008.09.003
[12] Bianco, P., & Robey, P. (2001). Stem cells in tissue engineering. Nature, 414, 118–121. https://doi.org/10.1038/35102181
[13] Hoang, D. M., Pham, P. T., Bach, T. Q., et al. (2022). Stem cell-based therapy for human diseases. Signal Transduction and Targeted Therapy, 7, 272. https://doi.org/10.1038/s41392-022-01134-4
[14] Acharya, A. B., & Wroten, M. (2023, February 13). Broca aphasia. In StatPearls [Internet]. StatPearls Publishing. Available from https://www.ncbi.nlm.nih.gov/books/NBK436010/
[15] National Aphasia Association. (n.d.). Aphasia statistics. National Aphasia Association. https://aphasia.org/aphasia-resources/aphasia-statistics/#:~:text=About%20750%2C000%20strokes%20occur%20each,in%20the%20USA%20with%20aphasia
[16] National Aphasia Association. (2022). 2022 Aphasia Awareness Survey. National Aphasia Association. https://www.aphasia.org/aphasia-resources/aphasia-statistics/
[17] Damico, J. S., Müller, N., & Ball, M. J. (Eds.). (2021). The handbook of language and speech disorders. John Wiley & Sons. https://doi.org/10.1002/9781119606987
[18] Fridriksson, J., Fillmore, P., Guo, D., & Rorden, C. (2015). Chronic Broca's Aphasia Is Caused by Damage to Broca's and Wernicke's Areas. Cerebral cortex (New York, N.Y. : 1991), 25(12), 4689–4696. https://doi.org/10.1093/cercor/bhu152
[19] Katagiri, T., & Watabe, T. (2016). Bone Morphogenetic Proteins. Cold Spring Harbor perspectives in biology, 8(6), a021899. https://doi.org/10.1101/cshperspect.a021899
[20] Wuensch, C. (2019). Do humans possess the capability to regenerate? The Science Journal of the Lander College of Arts and Sciences, 12(2), Spring 2019.
[21] Vincent, E., Villiard, E., Sader, F., Dhakal, S., Kwok, B. H., & Roy, S. (2020). BMP signaling is essential for sustaining proximo-distal progression in regenerating axolotl limbs. Development (Cambridge, England), 147(14), dev170829. https://doi.org/10.1242/dev.170829
[22] Bond, A. M., Peng, C. Y., Meyers, E. A., McGuire, T., Ewaleifoh, O., & Kessler, J. A. (2014). BMP signaling regulates the tempo of adult hippocampal progenitor maturation at multiple stages of the lineage. Stem cells (Dayton, Ohio), 32(8), 2201–2214. https://doi.org/10.1002/stem.1688
[23] Schleidgen, S., Dederer, H. G., Sgodda, S., et al. (2020). Human germline editing in the era of CRISPR-Cas: Risk and uncertainty, inter-generational responsibility, therapeutic legitimacy. BMC Medical Ethics, 21, 87. https://doi.org/10.1186/s12910-020-00487-1
Comments